The ALS User Guide provides step-wise instructions for obtaining access to ALS research facilities, preparing for your visit, and completing post-visit follow-up. By following the guidance below, you can help ensure a successful and safe experience at our facility.
If your proposal is awarded beamtime, all on-site experimenters who are new users or users with an inactive status must register in advance: three weeks in advance for U.S. citizens and six weeks in advance for non-U.S. citizens.
Please visit this page for COVID-19 updates.
Get started:
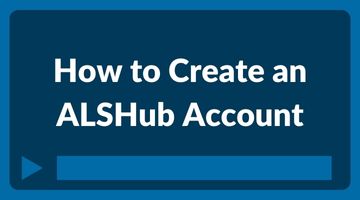
Prepare for your experiment:
4. Comply with experiment safety requirements
6. Complete online safety training
7. Utilize available resources
After your visit:
8. Complete the user satisfaction survey
9. Report Publications, Awards, and Talks Acknowledging ALS Work